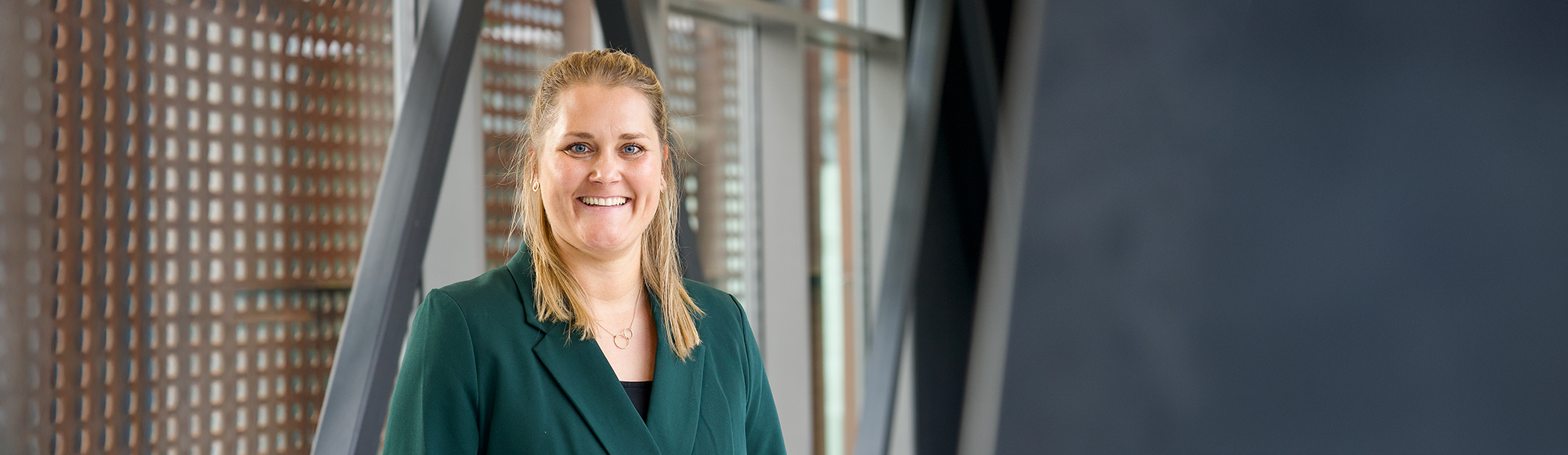
Exploring Earth-abundant manganese’s potential to replace rare and expensive metals
With a DKK 16.5 million grant, chemist Christina Wegeberg sets out to explore the potential of the Earth-abundant metal manganese to harvest sunlight. If she succeeds, manganese may serve as an alternative to the rare and expensive noble metals used today.
There are currently 118 known elements on Earth. Some are very common, surrounding us in abundant amounts, such as oxygen, hydrogen, nitrogen, and carbon. Others, like the noble metals ruthenium, iridium, and osmium, are extremely rare, difficult to mine, and highly sought after by industry.
As demand for these rare metals keeps rising, their low availability may pose significant challenges for the global supply chain in the future.
The reason Earth-abundant metals like iron, chromium, and manganese aren’t used as substitutes for the precious metals is that they don’t possess the same unique properties needed for certain applications.
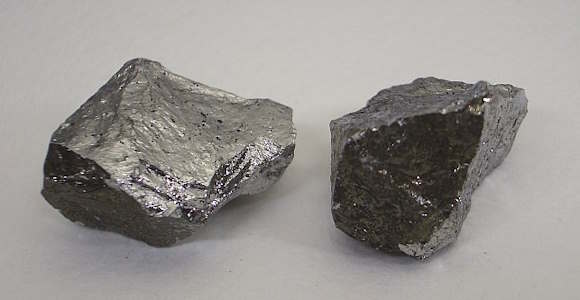
But why not explore whether these more common metals could be "taught" to act like their rare and costly counterparts, thus reducing dependency on rare elements that may only be found in a few potentially problematic places in the world?
This is precisely what Christina Wegeberg, assistant professor in chemistry, intends to investigate. With support from the Novo Nordisk Foundation, she will establish her own research group at SDU and closely study manganese to determine whether it is possible to use it for light harvesting and hereby potentially for solar energy conversion .
- Manganese is one of the most common transition metals on Earth. I believe manganese has untapped potential, with properties we can harness to capture sunlight and convert it into electrical or chemical energy. Imagine if we could replace noble metals like ruthenium, iridium, and osmium with manganese—that would definitely lead to a more sustainable solution, she says.
Capturing sun light
If she succeeds, it could open up a range of applications, from thin-film solar cells to synthetic pharmaceutical production.
- I’m not focusing too much on which specific applications my research might contribute to just yet. Right now, my main goal is to see if it’s at all possible to use manganese compounds to capture light. We can later determine the best applications once we succeed, says Wegeberg.
Her objective with manganese is to develop a manganese-based material that can harvest light energy. The hope is that sunlight (or LED light, laser light, or other light sources) could shine on a manganese-based material, and the manganese within its structure would absorb the light, converting it to chemical or electrical energy that could then power other processes. In other words, light should trigger an energy-generating process in the manganese compound.
Nature is the expert
This principle is called photoactivation, and Nature is a true master at it—as seen in photosynthesis, where plants capture sunlight and convert it into energy for growth.
The first step in this challenge is to create the proper framework in which manganese’s photoactivation can take place. Manganese mined directly can’t accomplish this on its own. According to Wegeberg, such a framework requires rigid skeletons of other elements like carbon, nitrogen, and hydrogen, known as ligands. These ligands must be rigid enough to hold the manganese atom entirely still, preventing it from losing energy through molecular vibrations.
The hope is that the ligands will keep the manganese compound in an excited state long enough to harvest the electrons released when the manganese compound is activated by light. These electrons could then be transferred to an electric circuit to generate current or be transferred to an organic molecule to initiate a reaction of a specific chemical. This could, for example, be used in drug manufacturing.
The best framework for manganese
This is the hypothesis. Now, Christina Wegeberg’s experiments will determine whether it’s feasible. To measure how long the manganese compounds can remain in the excited state, she will need advanced laser equipment, which the Novo Nordisk grant now enables her to acquire.
If Wegeberg’s research group one day can record an excited state lifetime measured in nanoseconds, it will be a success—in the world of photoactivation, the timescale of nanoseconds is long (one nanosecond is 0.000000001 seconds).
This duration is critical because molecules diffuse on the nanosecond timescale. By comparison, a non-optimal manganese framework would only result in excited state lifetimes of femtoseconds (one femtosecond is 0.000000000000001 seconds).
Research stay in Switzerland
Previously, while at the University of Basel in Switzerland, Wegeberg has worked on the element chromium, attempting the same goal: photoactivation of an Earth-abundant element. Link to paper.
The chromium project succeeded (achieved an excited state lifetime of 50 nanoseconds), and now, as she begins her own research group, she wants to focus on manganese.
- Manganese lies between chromium and iron in the periodic table, and they are placed in the row that has my interest because many of these transition metals are Earth-abundant. I’ve worked on chromium in Switzerland, other research groups worldwide are working extensively on iron, and now I’m setting out to tackle manganese. Zinc is also in this row of the periodic table and could likewise be interesting to explore someday, says Wegeberg.
”Imagine if we could replace ruthenium, iridium and osmium with manganese
If she succeeds in harnessing manganese to convert light to electrical energy, the sustainability impact would extend beyond simply using an Earth-abundant element, reducing dependency on rare elements that are more costly and environmentally damaging to extract. As she says:
- It must in general be far better to base our technologies on elements that are readily available here on Earth.
Developing light-activated materials could also enable the industry to perform challenging types of synthetic reactions under mild reaction conditions by harnessing energy from light. These milder reaction conditions open up entirely new possibilities for modifying biomolecules, while the higher energy input could enable the breakdown of unwanted chemicals that are otherwise difficult to dispose, such as in water purification applications.
Meet the researcher
Christina Wegeberg is an assistant professor and research leader at the Department of Physics, Chemistry, and Pharmacy. She has just received a RECRUIT grant from the Novo Nordisk Foundation for DKK 16.5 million to establish her own research group.